PCR vs qPCR vs RT-PCR vs RT-qPCR: What’s the Difference
- Molsentech
- Apr 29
- 10 min read
Updated: May 15
Polymerase Chain Reaction (PCR) is a fundamental laboratory technique used to amplify DNA sequences, making it easier to study specific genes or regions of interest. Over time, several variations of PCR have been developed to address different research and diagnostic needs. Follow our guide as we break down these variations simply—PCR, qPCR, RT-PCR, RT-qPCR, and dPCR—to help you select the appropriate method for a given application. Let’s go!
What is PCR (Polymerase Chain Reaction)?
PCR, also known as end-point, traditional, or conventional PCR, is a widely used laboratory technique that enables the amplification of specific DNA sequences, producing millions of copies from a small initial sample. This process involves three main steps:
Denaturation: The reaction mixture is heated to around 94–98°C, causing the double-stranded DNA to separate into two single strands.
Annealing: The temperature is lowered to approximately 50–65°C, allowing short DNA primers to bind, or anneal, to their complementary sequences on the single-stranded DNA templates.
Extension: The temperature is then raised to about 72°C, the optimal temperature for DNA polymerase to synthesize new DNA strands by adding nucleotides to the annealed primers.
These steps are repeated for 20–40 cycles in a device called a thermal cycler, leading to exponential amplification of the target DNA sequence.
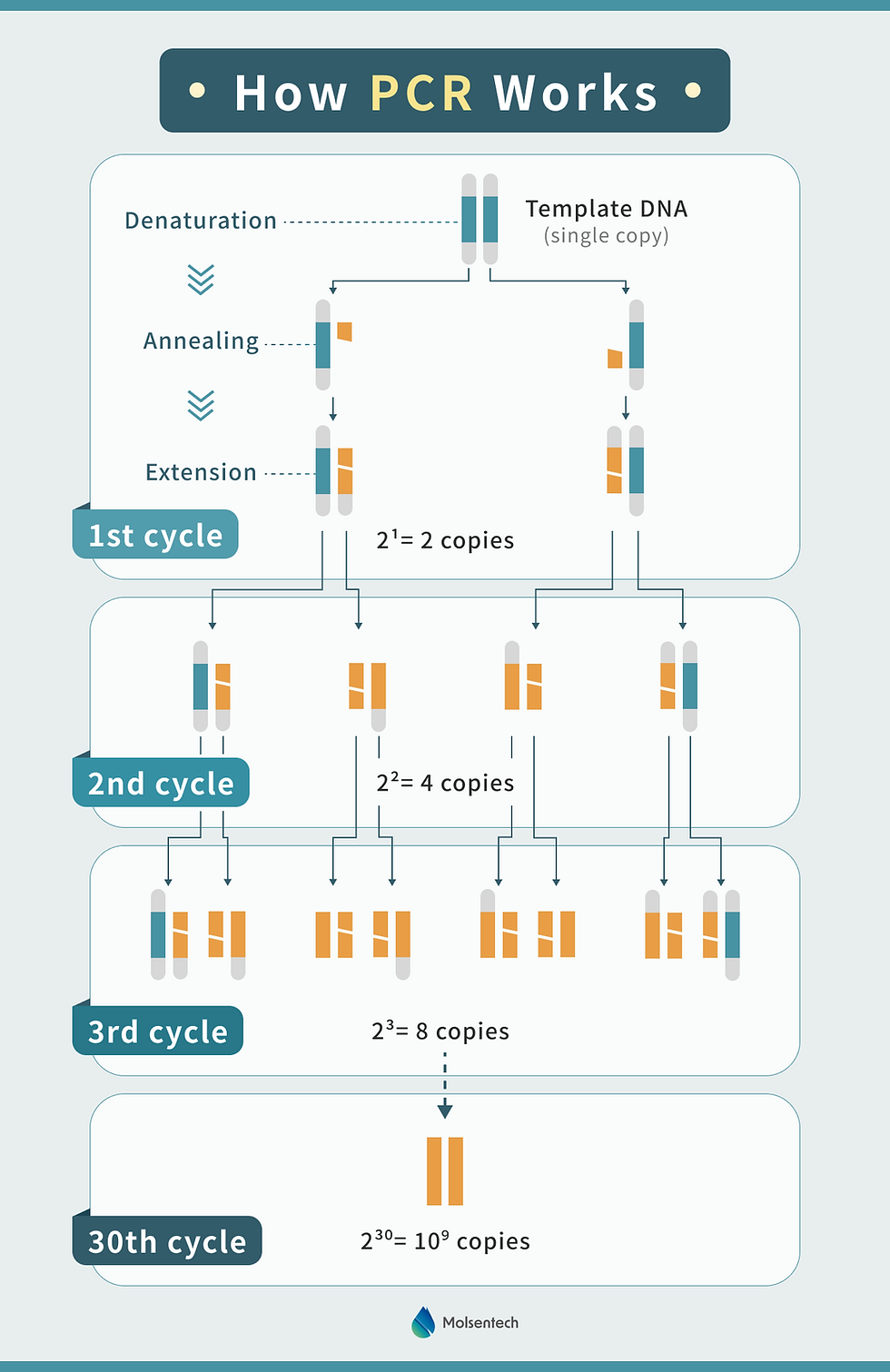
Developed by Kary Mullis in 1983, PCR has revolutionized molecular biology by allowing scientists to study specific DNA regions without the need for large initial samples. The essential components for PCR include a thermal cycler to precisely control temperature changes, DNA primers, nucleotides, DNA polymerase (commonly Taq polymerase), and the DNA template to be amplified.
The method is fundamental in various fields, including genetic research, forensic science, and clinical diagnostics, making detailed analysis tasks such as gene cloning, pathogen detection, and genetic mutation analysis more feasible.
Hot Start PCR
Hot start PCR is a refined version of the standard PCR technique designed to enhance specificity and yield.
In traditional PCR, the DNA polymerase can become active at lower temperatures during the setup phase, leading to non-specific amplification and the formation of primer dimers. Hot start PCR addresses this issue by keeping the DNA polymerase inactive until the initial denaturation step, typically achieved by using chemically modified enzymes or antibodies that block the polymerase's activity at room temperature.
Once the reaction mixture is heated during the denaturation phase, these modifications are reversed, activating the polymerase. This controlled activation prevents unwanted amplifications, resulting in cleaner and more accurate PCR outcomes.
What is qPCR (Quantitative PCR) / Real-time PCR?
Quantitative PCR, commonly known as qPCR or real-time PCR, is a laboratory technique that amplifies and quantifies DNA sequences simultaneously.
Unlike traditional PCR, which only indicates the presence or absence of DNA at the end of the process, qPCR measures DNA concentration during the amplification process. This is achieved by using fluorescent dyes or probes that emit light when bound to double-stranded DNA. As the DNA amplifies, the fluorescence increases proportionally, allowing for real-time monitoring.
This method allows scientists to measure the amount of DNA present at each cycle, providing both qualitative and quantitative data. qPCR is highly sensitive and specific, making it a valuable tool in various applications, including gene expression analysis, pathogen detection, and genetic variation studies. Its ability to provide rapid and accurate quantification of DNA makes qPCR an essential technique in molecular biology and medical diagnostics.
What is RT-PCR (Reverse Transcription PCR)?
RT-PCR, or reverse transcription polymerase chain reaction, is a technique that allows scientists to study RNA by converting it into DNA.
This process begins with the enzyme reverse transcriptase, which transforms RNA into complementary DNA (cDNA). Once converted, the cDNA undergoes amplification through PCR, enabling a further detailed analysis. RT-PCR is particularly valuable for detecting RNA viruses and examining gene expression.
Its ability to amplify specific RNA sequences makes it a powerful tool in research and diagnostics. By providing insights into gene activity and the presence of RNA-based organisms, like viruses, RT-PCR has become essential in molecular biology.
What is RT-qPCR (Reverse Transcription Quantitative PCR)?
RT-qPCR, or reverse transcription quantitative PCR, is a powerful technique that combines reverse transcription of RNA into cDNA with quantitative PCR. Note that the “RT” here does not stand for “Real Time.”
This method enables the detection and quantification of RNA targets, making it invaluable for analyzing gene expression and detecting RNA viruses. The process begins with reverse transcription, where RNA is converted into cDNA using the enzyme reverse transcriptase. Subsequently, the cDNA serves as a template for quantitative PCR, which amplifies the target sequence and measures its quantity in real time through fluorescence detection.
RT-qPCR offers high sensitivity and specificity, allowing for the detection of low-abundance RNA molecules. Its quantitative nature provides precise measurement of gene expression levels, providing insights into cellular functions and disease mechanisms. It’s also a go-to method for detecting RNA viruses as well, like SARS-CoV-2, which makes it incredibly useful in diagnosing and managing diseases.
What is dPCR (Digital PCR)?
Digital PCR (dPCR) is a cutting-edge method for detecting and measuring DNA and RNA.

The process of digital PCR (dPCR) starts with preparation, where DNA or RNA is extracted from the sample, purified, and mixed with reaction components. These include primers, nucleotides, DNA polymerase, and fluorescent probes or dyes.
The prepared mixture then undergoes partitioning, where the sample is divided into thousands of tiny, isolated reactions using specialized equipment, such as microfluidic chips or droplet generators. Each partition contains zero, one, or a few target DNA molecules. This unique approach makes it easier to pinpoint and count target molecules with incredible accuracy.
During amplification, the partitions are subjected to thermal cycling, similar to traditional PCR, to amplify the target DNA within each partition. Fluorescence is generated in positive partitions where the target is amplified.
Finally, in the analysis phase, the number of positive partitions is counted, and statistical calculations are applied to determine the absolute quantity of the target DNA or RNA in the original sample.
One of the standout features of dPCR is its ability to provide absolute quantification. This means it can measure the exact number of DNA or RNA molecules without relying on comparison to a standard curve, which is required in other methods like qPCR.
This makes it perfect for situations where precision matters most. Also, it’s much less affected by substances in the sample that might otherwise mess up results. Even in tricky samples, like those from blood or environmental sources, dPCR remains reliable and consistent.
Digital PCR does come with its own set of requirements. It needs specialized machines to create these tiny compartments and to measure their results. This adds complexity compared to traditional PCR techniques. But for applications where high sensitivity, accuracy, and reliability are crucial, dPCR is unmatched.
PCR vs qPCR vs RT-PCR vs RT-qPCR vs dPCR: Compare the Differences
Understanding the nuances between PCR, qPCR, RT-PCR, RT-qPCR, and dPCR is essential for tailoring molecular biology techniques to specific research and diagnostic needs. Each method has unique strengths and applications. Let’s dive deeper.
Starting Material
Traditional PCR and qPCR both use DNA as their starting material, making them ideal for amplifying and quantifying specific DNA sequences. RT-PCR and RT-qPCR begin with RNA, which is converted into cDNA through reverse transcription, enabling the analysis of RNA viruses and gene expression.
Digital PCR (dPCR), on the other hand, is flexible and can use either DNA or RNA (after reverse transcription), offering absolute quantification regardless of the starting material, with unmatched precision.
Quantification
Quantification is essential for applications like gene expression analysis and viral load assessment, where precise nucleic acid measurements are critical. PCR techniques differ significantly in their quantification abilities.
Traditional PCR only detects DNA presence, without quantification. qPCR tracks DNA amplification in real time, enabling relative quantification. Similarly, RT-PCR detects RNA but does not measure its quantity. RT-qPCR enhances this by combining RNA analysis with real-time quantification for precise results.
Digital PCR (dPCR) excels in absolute quantification, directly counting nucleic acids without standard curves. This approach offers unmatched precision, making it ideal for detecting low-abundance targets.
Sensitivity
Sensitivity is crucial in PCR techniques because it determines the ability to detect even the smallest amounts of nucleic acids in a sample. High sensitivity is essential for diagnosing diseases, identifying rare genetic mutations, or working with minimal sample sizes.
Traditional PCR has moderate sensitivity, adequate for many tasks but less effective for rare or low-level targets. qPCR improves on this, offering higher sensitivity with real-time detection of small amounts of DNA.
RT-PCR provides moderate sensitivity for RNA detection but lacks precision in quantification. RT-qPCR combines high sensitivity with quantification, making it a robust choice for low-abundance RNA analysis.
dPCR, however, stands out with the highest sensitivity, enabling the detection of extremely rare targets and low-frequency mutations, making it invaluable for applications requiring utmost accuracy in detection.
Precision
Precision refers to the ability of a PCR technique to consistently provide accurate and reproducible measurements. It ensures that results are reliable, especially for experiments requiring detailed quantification, such as subtle gene expression studies or low-frequency mutation detection.
Traditional PCR is reliable for amplifying nucleic acids but lacks precision for quantification. qPCR enhances precision by offering real-time quantitative data, though results can depend on standard curves.
RT-PCR focuses on RNA detection and is qualitative, lacking emphasis on precision. RT-qPCR improves accuracy in RNA quantification by combining reverse transcription with real-time analysis.
dPCR leads in precision, as it partitions samples into thousands of reactions, eliminating reliance on standard curves and reducing variability. This makes it ideal for highly precise tasks, such as rare mutation analysis and absolute nucleic acid quantification.
Ease of Use
Ease of use is key to making laboratory techniques accessible and efficient. It directly impacts workflow, training requirements, and the feasibility of implementing different methods in various settings.
Traditional PCR is the easiest, requiring basic equipment and simple protocols. This makes it a go-to option for most labs. qPCR introduces real-time fluorescence monitoring, which calls for specialized machines and some technical know-how.
RT-PCR adds a reverse transcription step, making it a bit more involved. RT-qPCR combines reverse transcription with real-time monitoring, further increasing complexity and requiring advanced skills and equipment.
Digital PCR (dPCR) is the most complex. It uses specialized instruments for sample partitioning and detailed data analysis. Although it provides unmatched accuracy and sensitivity, it is less user-friendly than other methods.
Cost
Cost is an important factor when choosing a PCR technique, especially for labs with budget constraints.
Traditional PCR is the most budget-friendly option, requiring basic reagents and equipment. qPCR is pricier due to its need for fluorescent probes and real-time detection systems. RT-PCR costs more than PCR, as it includes reverse transcription reagents.
RT-qPCR combines the expenses of reverse transcription and real-time quantification, making it even more costly. Digital PCR (dPCR) tops the chart as the most expensive, thanks to its advanced partitioning technology, high-end equipment, and consumables.
Large Volume Tested Samples
When working with large-volume samples, the ability of a PCR technique to handle the sample size without introducing errors is crucial. If the instrument processes only small volumes, rare targets may not be adequately captured, leading to inaccurate quantification.
Traditional PCR and RT-PCR can handle moderate sample volumes but are limited to qualitative analysis. qPCR and RT-qPCR excel in accommodating larger sample volumes, making them ideal for detecting low concentrations of nucleic acids in bulk samples.
On the other hand, digital PCR (dPCR), though highly precise, often processes smaller volumes due to its partitioning approach, making it less suitable for such applications.
Uses
Traditional PCR is versatile for tasks like gene cloning, mutation detection, and genotyping. qPCR shines in quantifying gene expression, detecting pathogens, and analyzing genetic variations.
RT-PCR is widely used for studying RNA, such as detecting RNA viruses or examining gene expression. RT-qPCR combines these applications with precise RNA quantification, making it ideal for monitoring viral loads or measuring gene activity.
Digital PCR (dPCR) stands out in applications requiring absolute precision, like detecting rare mutations, analyzing complex samples, or performing liquid biopsies in cancer research.
Factor | PCR | qPCR | RT-PCR | RT-qPCR | dPCR |
Starting Material | DNA | DNA | RNA (converted to cDNA) | RNA (converted to cDNA) | DNA or RNA (via cDNA) |
Quantification | No | Yes (real-time, relative) | No | Yes (real-time, relative) | Yes (absolute, exact counts) |
Sensitivity | Moderate | High | Moderate | High | Very High |
Precision | Reliable for presence/absence | High for real-time data | Reliable qualitative | High for RNA quantification | Extremely high for rare targets |
Ease of Use | Simple | Moderate complexity (fluorescent probes) | Requires reverse transcription | Advanced (combines RT and real-time quantification) | Advanced (partitioning samples required) |
Cost | Low | Moderate to high | Moderate | High | Very high |
Sample Volume Capacity | Moderate | Large | Moderate | Large | Small |
Uses | Cloning, genotyping, mutation detection | Gene expression, pathogen detection | RNA virus detection, gene expression | Viral load, RNA quantification | Rare mutation detection, absolute quantification |
Other DNA Amplification Techniques
While PCR is a cornerstone in DNA amplification, other techniques like isothermal amplification, whole genome amplification, and bridge amplification have unique advantages. Let’s explore these methods, comparing their strengths and limitations alongside the features of PCR.
Isothermal Amplification
Isothermal amplification is a method that amplifies DNA at a constant temperature, unlike PCR, which requires cycling through different temperatures.
Techniques like Loop-Mediated Isothermal Amplification (LAMP) fall under this category. These methods are faster and often more portable since they don’t need thermal cyclers. For example, LAMP can amplify DNA in under an hour and is useful for field-based applications like disease diagnosis in remote areas.
However, isothermal amplification is less specific than PCR, which can lead to false positives. While isothermal methods are great for speed and simplicity, PCR's precision and flexibility in amplifying specific DNA sequences give it an edge for detailed laboratory analyses.
Whole Genome Amplification
Whole Genome Amplification (WGA) is designed to amplify an entire genome, rather than just a specific DNA region.
This technique is valuable when working with very small or degraded DNA samples, such as those from ancient artifacts or single cells. While WGA can recover vast amounts of genetic material, it can introduce biases, potentially over-representing certain DNA regions.
In contrast, PCR focuses on amplifying specific sequences with high fidelity, making it more accurate for targeted studies. WGA is ideal for applications requiring a broad genetic overview, while PCR excels in precision and targeted investigations.
Bridge amplification
Bridge amplification is a method used in next-generation sequencing (NGS). It involves binding single-stranded DNA to a surface where it forms a "bridge" with complementary adapters. This allows for cluster generation, creating millions of identical DNA copies for sequencing. It is highly efficient for sequencing workflows but is not versatile for other DNA analyses.
Compared to PCR, which is adaptable to various applications like diagnostics and cloning, bridge amplification is limited to NGS contexts. While bridge amplification ensures high-throughput sequencing, PCR remains the gold standard for its broad application range and accessibility in most molecular biology labs.
An Alternative to PCR: Molsentech’s Amplification-Free Technology
PCR and its advanced variations have revolutionized molecular biology by enabling the amplification and analysis of nucleic acids. However, these methods often require intricate workflows, costly reagents, and specialized equipment. Amplification, while powerful, adds complexity and time to diagnostic processes. Molsentech is breaking these barriers with its Bio-FET chip technology, which eliminates the need for amplification altogether.
Molsentech, a pioneering biotech startup from Taiwan, is redefining diagnostics with its cutting-edge Bio-FET platform. By harnessing nanotechnology, advanced chemistry, AI algorithms, and automation, our system delivers ultra-sensitive, rapid, and precise detection of biomolecules—transforming healthcare diagnostics.
During the COVID-19 pandemic, Molsentech achieved a historic milestone as their nucleic acid detection chip received Taiwan’s Emergency Use Authorization (EUA), showcasing our effectiveness in real-world applications. Beyond COVID-19, this platform targets challenging diseases like Alzheimer’s, cancer, and infectious diseases, enabling earlier diagnosis and life-saving interventions.
We are revolutionizing healthcare with innovative technologies that save lives. Contact us for more information about our technology and experience the future of diagnostics today.
Comments